Getting the most out of energy harvesting
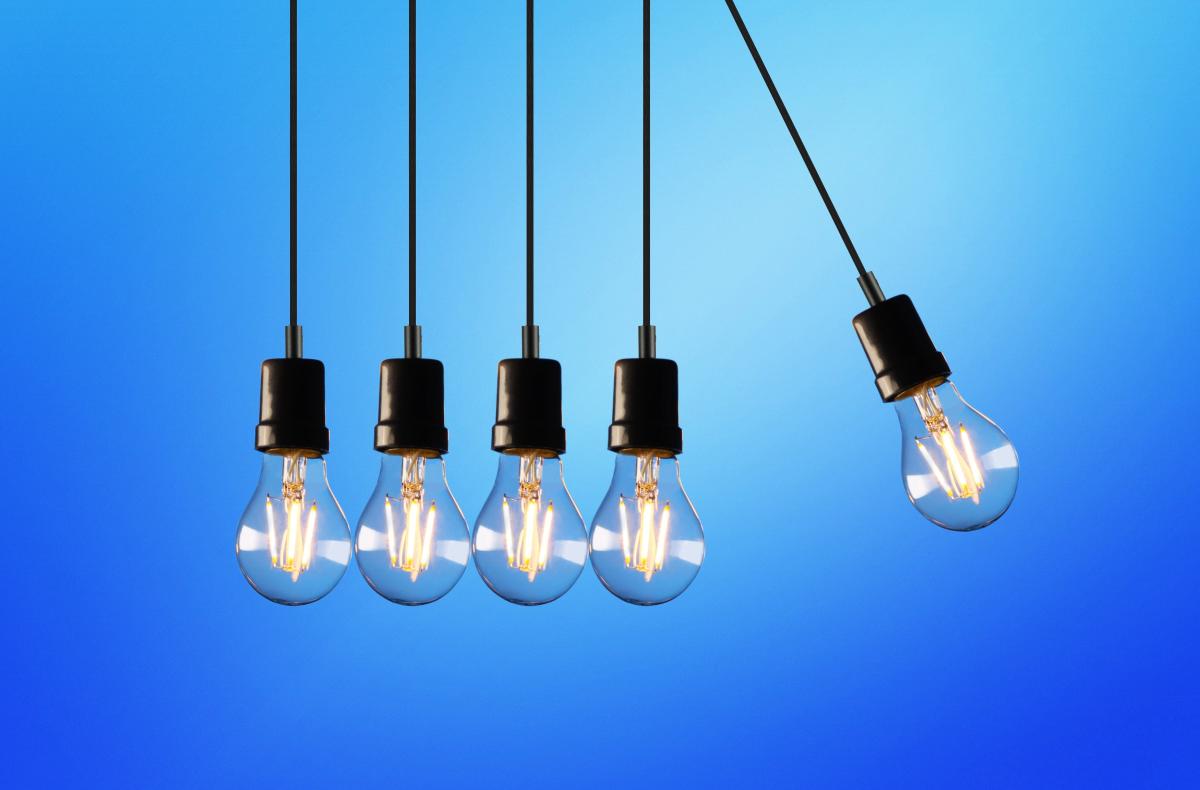
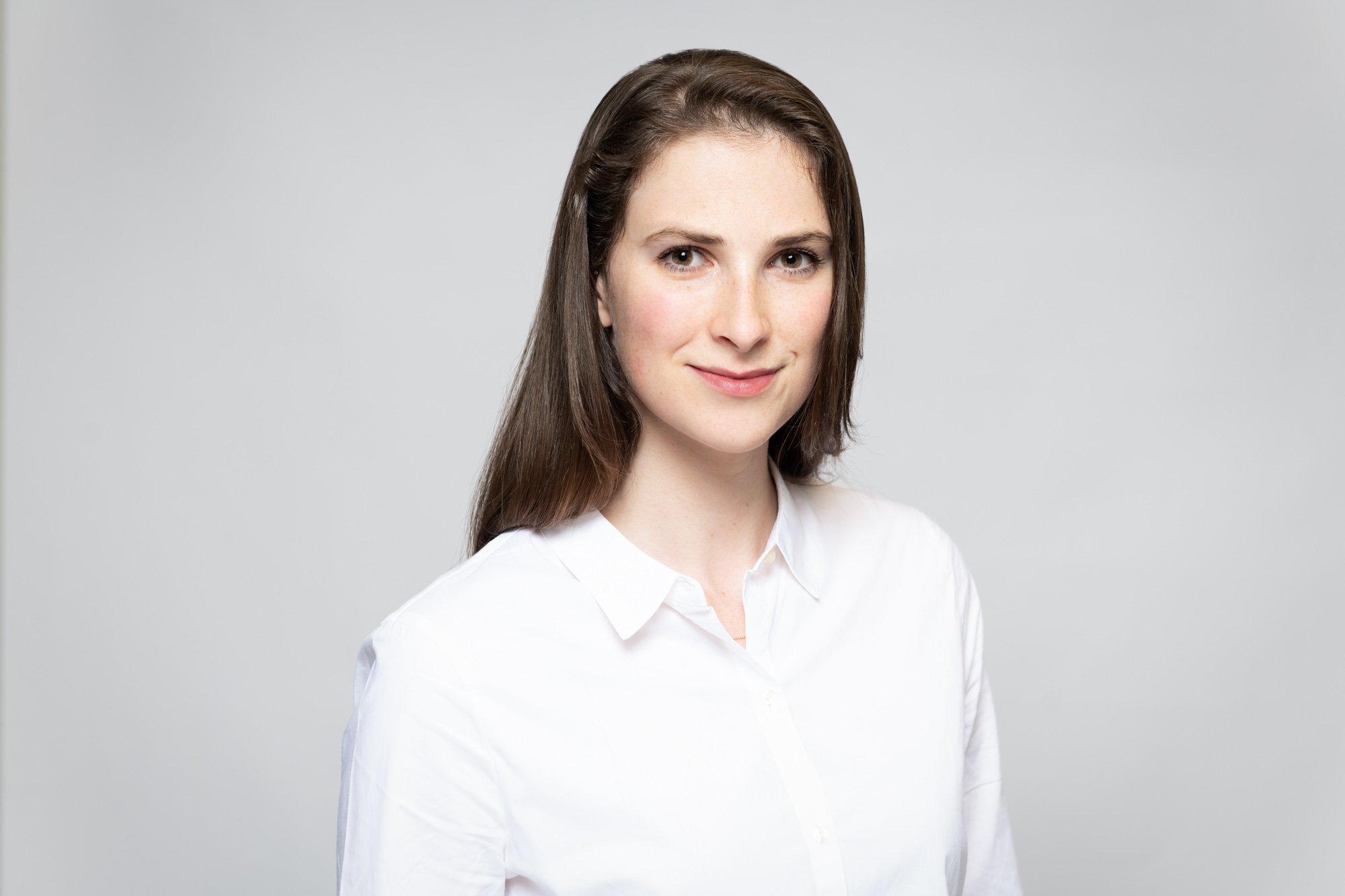
The growing Internet of Things (networked devices such as smart sensors) runs on data. Sensing, communicating, and collecting large amounts of data is at the core of what makes IoT useful: networks measure data (whether recording human activities, sensing environmental conditions, or something else), feed those measurements into the central system or make use of data locally, and perhaps take action in response to the information they have. But the energy demands of these networks over their lifetimes are considerable. That means that powering these systems is a major bottleneck for scalability, long network lifetimes, and sustainability.
Traditional battery-based systems have a continuous and stable energy supply… until the batteries run out. As the number of connected IoT devices grows, the need for regular battery replacement implies a growing cost burden (not only financially, but also environmentally), which may be unacceptable. Plugging many small devices into the building’s wiring entails similar problems, as the number of sockets needed escalates, and for that matter the wires themselves are expensive, bulky, and aesthetically displeasing. In other words, installation is a major pain point.
An alternative design is for IoT nodes to harvest energy from their environment (for example, with a small solar panel) to power themselves. But what seems like a perfect solution is anything but simple.
A good harvest needs enough light
In the typical office environment, natural light from windows may not penetrate far into the space, and some areas (such as storerooms) will have no natural light at all. Meanwhile, artificial light appears and disappears at the flick of a switch. This means that even though two IoT nodes might be only a few metres apart, they can have widely different energies available, harvesting more or less and at different times. They can store some energy to keep running overnight, or maybe over a weekend, but they still adapt their behaviour to their own changing environments, which can affect the network as a whole.
Think of an office building retrofitted with automated temperature and air quality controls. The control system comprises a network of solar-powered energy harvesting nodes installed throughout the building, together with an always-powered central control. The nodes measure the local temperature and air quality and communicate the measurements to the central control, which sets the heating and ventilation in response to this gathered data to ensure a comfortable and healthy climate.
Because the sensors rely on light for power, they are limited in when and how often they can measure and provide information. But there are times when getting frequent updates is more important (such as during large meetings, when the air quality is worsening) and times when it is less important, such as overnight when the building is empty.
Data gathering isn’t the only challenge; efficient communication is another. Communication between nodes and the central system over long distances, such as between different rooms of a building, requires a lot of energy – but ensures that it is always possible for nodes to communicate with the central system. As an alternative, in multi-hop communication, nodes rely on other nodes to pass along their data. Each node sends its message to nodes that are nearby, which then passes it on again, and so on until the message reaches the correct recipient. But what if the nodes forwarding those data packets are out of energy?
Unpredictable conditions make it hard to predict and plan which nodes will have energy at what times (making them available to pass along data). This makes it hard for a node to adapt its behaviour, since it depends on others.
These are areas being explored by NCCR Automation researcher Naomi Stricker. She and her collaborators are devising strategies, co-designing hardware and software, and developing communication systems to overcome the challenges and enable energy harvesting systems in new applications.
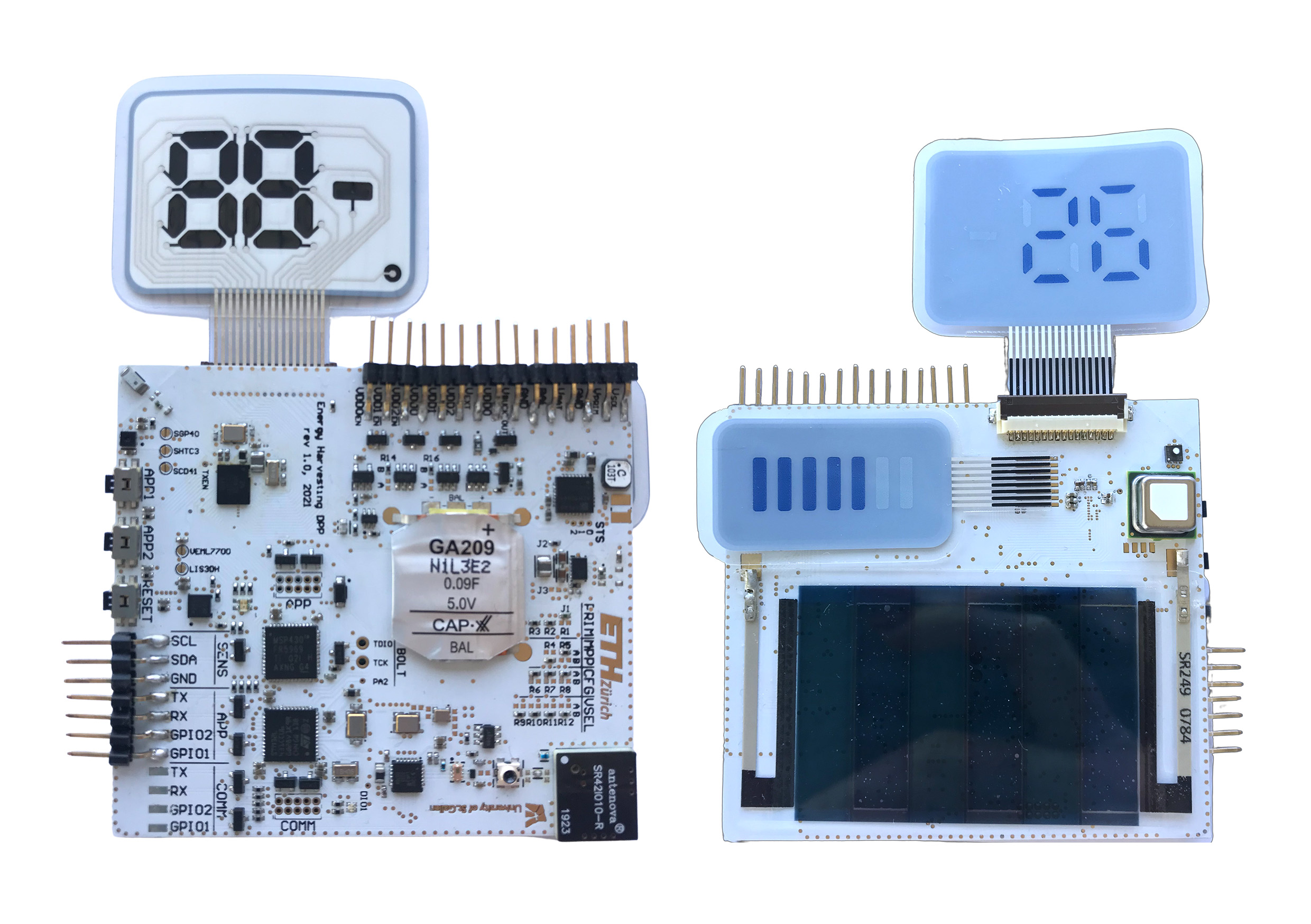
What you know, and when you need it
The key point, in designing parsimonious control systems with energy harvesting nodes, is the need to balance the following two factors: first, when and how often information is available (which of course is dictated by energy availability); and second, when that information is needed.
This means optimising measurement timings based on how much energy is available at the sensor nodes for future measurements, and how important that information is. In other words, the innovative algorithm assesses the long-term energy availability of the energy harvesting nodes and schedules the time for the next measurement by balancing this energy availability against the benefit the control system obtains from obtaining information at that point. Our new work in this area enables efficient integration of energy harvesting sensor nodes into control loops by considering both their energy limitations and the control quality.
Another aspect is communication. Our dynamic routing approach makes the most of the varying and variable resources available to nodes in a network, finding the most energy-efficient path depending on node availability. Intermediate nodes will only be sent data if they have already been found to be active; if not, long-range communication comes into play. In this case the communicating nodes will use more energy until more intermediate nodes come online.
By adapting to changing conditions, the system can avoid both wasting energy on long-range data transfers, and allowing communication failures and delays when some nodes are out of service. (If only as humans we could also be sure our audience was listening before we spoke.)
Taking a holistic view of the energy harvesting challenges means that we can approach diverse topics from many sides. Our research has led to a wider understanding of the reliability and robustness of energy harvesting nodes; to improvements in energy harvesting predictions; novel concepts for efficient communication in energy harvesting networks; and a framework for the use of energy harvesting sensor nodes in control systems.
Putting this all together can pave the way for new scalable networked applications, as well as making existing applications cheaper and lowering their environmental impact. This points the way to unlocking the full potential of energy harvesting IoT for sustainable cities and industrial development.
Written by Robynn Weldon and Naomi Stricker.